It's a very special delivery.
About once a month, researchers at the PARADIM Bulk Crystal Growth Facility place a small parcel in the mail. They wrap the precious cargo in bubble wrap and packing peanuts, apply a "fragile" sticker, and write "nontoxic mineral crystals" on top to describe its content. Postal service employees ferry the parcel across the country. Unbeknownst to them, they are transporting substances that—up until a few days ago—had never existed.
That bears repeating. The contents of these boxes had never occurred in the 13.7 billion-year history of the universe. Until now.
Produced on the ground floor of the Bloomberg Center for Physics and Astronomy on the Homewood campus of Johns Hopkins University, these materials will make up the next generation body armor, solar panels, quantum computers, sensors, and lasers.
New. Exotic. Filled with possibilities. That's the business of the Bulk Crystal Growth Facility.
"Most people don't typically think about this, but materials underlie everything in our life," explains Tyrel McQueen, the director of the facility. "Everything is built out of something. Materials are made of atoms and the reason they behave the way they do is all about the way in which these atoms are arranged."
He brings up bone, his favorite example. "Bone is a remarkable material because it is simultaneously strong and flexible. Normally things that are very strong are brittle—think of a ceramic dinner plate—whereas things that are malleable are soft and easy to bend. Bone somehow combines those two properties. And, we know why that is: It is because of the atomic structure of bone that is not random but highly structured."
McQueen's team goes about purposefully designing and synthesizing substances with desired properties. Outfitted with unique, cutting-edge equipment, his facility allows researchers to combine atoms in new ways to engineer the materials of tomorrow—materials that will make technologies faster, smarter, and greener.
The project got its start four years ago when McQueen joined forces with colleagues at Cornell, Princeton, and Clark Atlanta University. They proposed a national user facility giving researchers around the country access to unparalleled expertise and leading-edge equipment. Researchers propose projects and, through a competitive process, are invited to use the facilities. Funded by the National Science Foundation to the tune of $28 million, the collaboration is called PARADIM, short for the Platform for the Accelerated Realization, Analysis, and Discovery of Interface Materials.
"The users who come here may have a problem that we alone may be able to help with," says Lucas Pressley, a doctoral candidate working at PARADIM. "Or, where the users may be able to synthesize their new material in one to two years, we can do it in a week or a month."
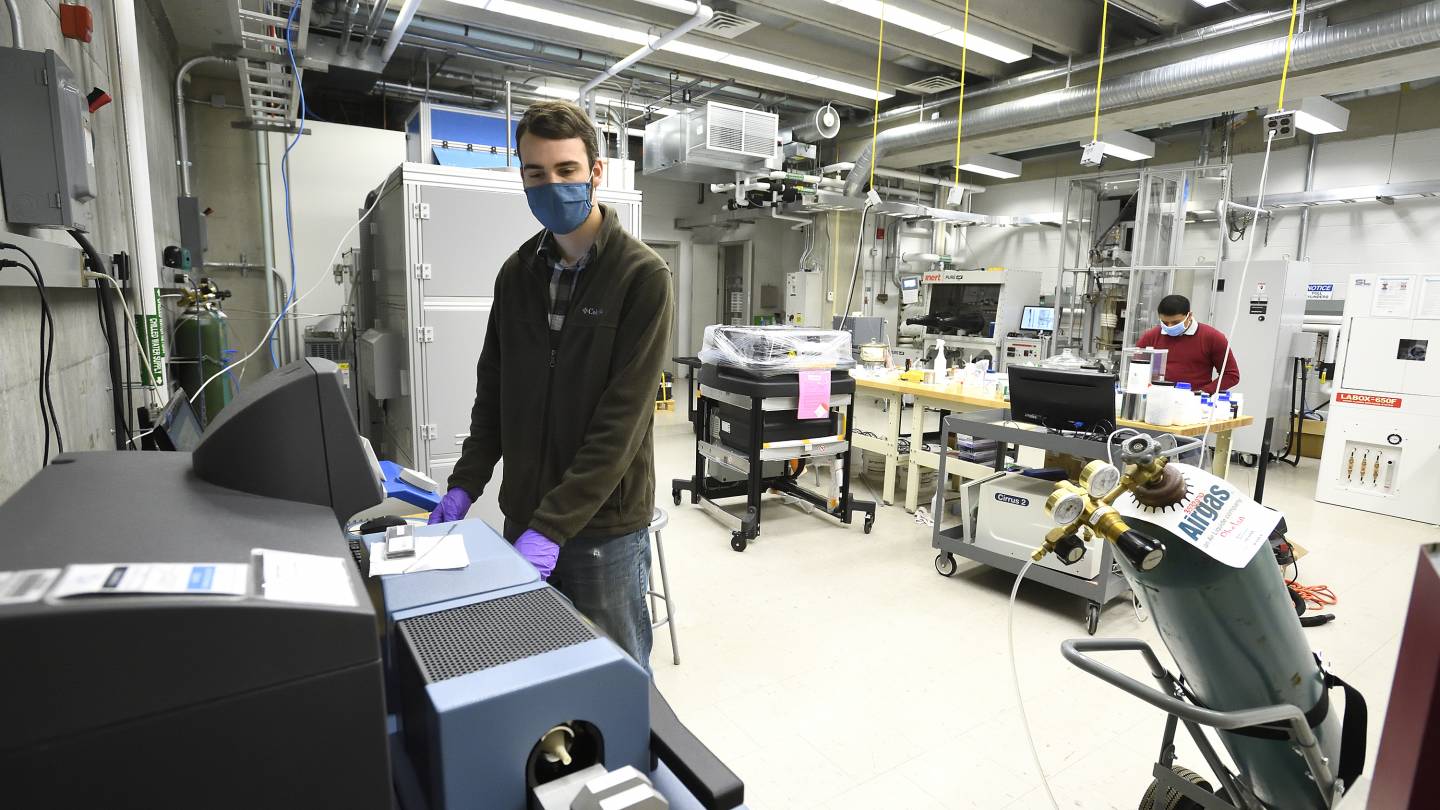
Image credit: Will Kirk / Johns Hopkins University
There are two established strategies for synthesizing new materials. The first is to lay down each atom in a controlled fashion—an atomic-scale 3D printer. But, there is a problem with this strategy. "There are a mind-bogglingly large number of atoms in a sample the size of a screwdriver," explains McQueen. "Even if positioning atoms is super fast, say once every [quadrillionth of a second], it is still going to take you in the order of the age of the universe to build a screwdriver by that approach." Clearly, while that level of control is enviable, it is not scalable.
The other option is to take large quantities of starting materials and melt them. This is the process used to make steel. "That gives you a lot of control at the scale of the screwdriver, but that doesn't allow you to control the individual atoms," says McQueen.
This leaves an interesting gap. "How do you make something that's the size of a screwdriver with some level of atomic control?" asks McQueen. That's what PARADIM sets out to do.
It does it by growing crystals. The atoms in a crystal organize themselves into an ordered, repeating pattern, and they do it rapidly. A common experience with this phenomenon is watching frost grow on a window. The pattern we observe with our eyes echoes what is happening at the atomic scale.
"The analogy I really like is that of a cornfield where you can see uniform rows going in one direction," explains Pressley. "You can think of those plants arranged in rows as the arrangement of atoms if they are in a single crystal." This ordered array of atoms in a crystal makes it easier to study the material and can give rise to emergent properties. These novel properties may in turn be useful in building electronics, for example by capturing a device's waste heat and converting it into electricity.
So, how do researchers grow crystals?
"Ideally you would have a planetary simulator," laughs Pressley about the extreme conditions of heat and pressure required to disrupt the bonds between atoms in the starting material. Once melted, the material is slowly cooled, giving the atoms a chance to find new partners and arrange themselves into the ordered configuration of the crystal.
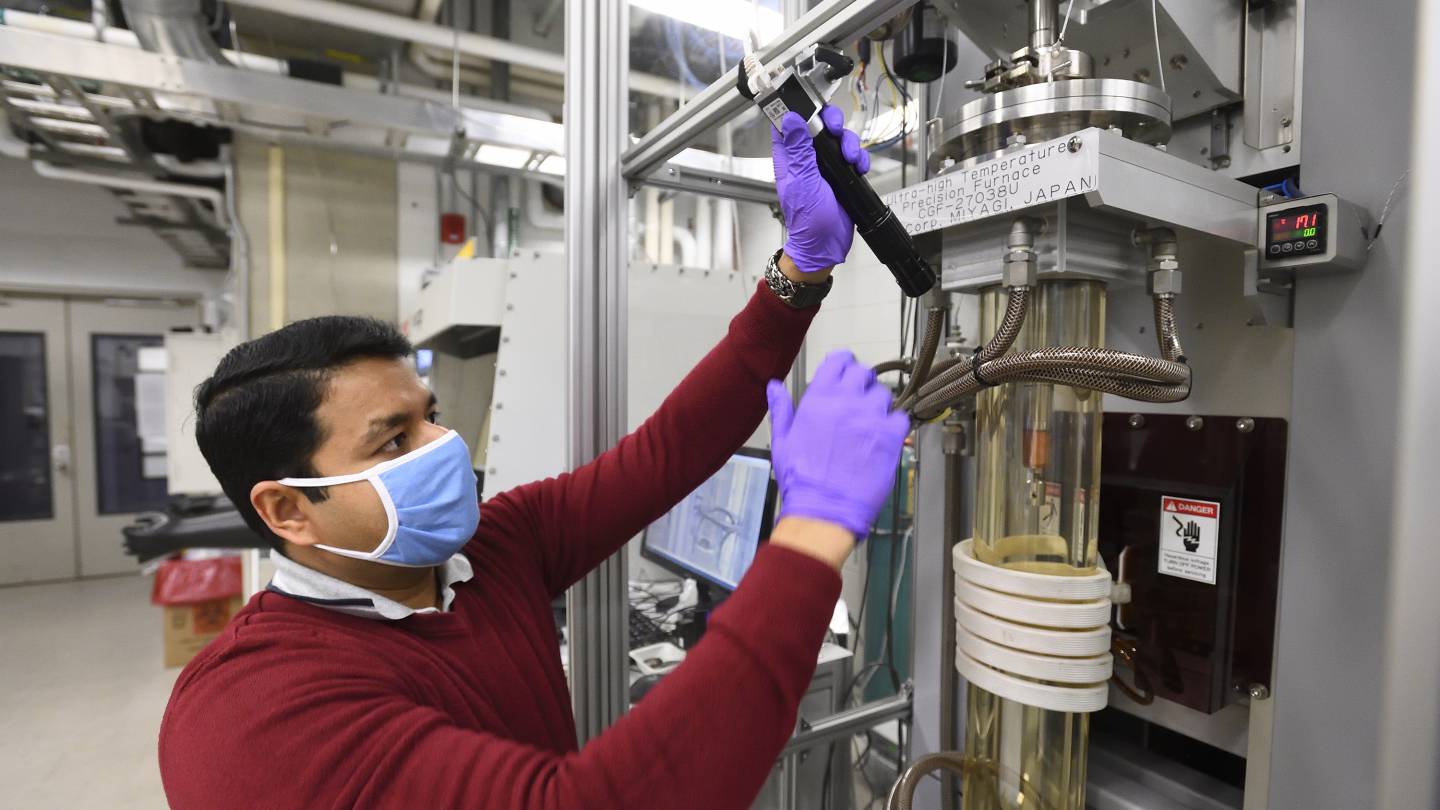
Image caption: Bulk crystal scientist Mojammel Khan loads a furnace in the PARADIM lab
Image credit: Will Kirk / Johns Hopkins University
At PARADIM, this means using furnaces—lots of specialized furnaces. Some aim focused laser beams at the starting compounds; others reflect the heat of powerful bulbs with mirrors; and others induce rapidly oscillating currents in the material to heat it up. Temperatures can reach 3,000°C (5,432°F)—that's twice the temperature of Hawaiian lava.
"It's very focused and confined heat," explains Mekhola Sinha, a doctoral student and PARADIM researcher who helped set up the facility four years ago. "We apply the heat to only a small area to heat that portion of the sample."
Researchers around the country flock to the Bulk Crystal Growth Facility to access these unique furnaces and learn how to use them. "The paradigm here is not just 'you send us a sample and we send you a crystal,'" Pressley says. "There is knowledge gained from working with us. You are learning the process and becoming a better scientist as a result of it."
But, in the wake of the COVID-19 pandemic, few people are around. The pervasive hum of the ventilation system that keeps the PARADIM equipment cool is now deafening. Remarkably, this has not stopped the activities of the facility.
"A lot of our equipment, since day one, was set up to be run remotely," McQueen says. "We have always offered this to users. They have almost universally declined and chosen to come and do it with their own two hands."
The furnaces are equipped with cameras and monitoring equipment that allow researchers anywhere in the world to see what is happening—and control it—in real time.
"Once the samples are loaded [in a furnace], you can go home and sit on your couch and run the crystal growth while watching TV," Pressley says.
The team came up with even more innovative ways to conduct its activities remotely. Each summer, PARADIM trains a cohort of researchers—students and professors—in the methods used to make and characterize new materials. The participants roll up their sleeves and try their hand at a one-week synthesis project.
"This couldn't happen this year," reports PARADIM doctoral trainee Nicholas Ng. "What we said to them is, I am going to wear a Go Pro camera on my head. You guys are going to see exactly what I see with a live feed. You are going to puppeteer me. You are going to drive my hands and tell me what to do. Tell me how much of each element to put in, what kind of tube to use, and the best course of action here."
"Our participants reported that the school was quite successful," McQueen says.
Ng, who enjoys working with the unique equipment, has prepared a pitch to attract colleagues to PARADIM. With a mischievous smile, he admits, "I tell them, 'Come hit your compounds with the power of the sun in a little box.'"
Posted in Science+Technology
Tagged tyrel mcqueen, physics and astronomy