About 4.6 billion years ago, in the Gould Belt of the Orion Arm of the Milky Way galaxy, a massive cloud of dust and gas collapsed into a spinning disk, possibly from a shock wave blasted out by a nearby supernova. In the center of this disk, gravity pulled together a ball of mostly hydrogen that as it condensed became hotter and hotter and more and more pressurized until hydrogen atoms began fusing into helium, which released tremendous energy. Ignition—the sun, our sun, was born.
Glance at it and you will not see the sun as it is now. You will see it as it was more than eight minutes in the past because it took the photons 8:20 to traverse the 93 million miles to Earth. But those photons did not beam straight out from the sun's core, where they were generated. They traveled only a millimeter or so toward the visible surface before they were absorbed and then radiated again, absorbed and radiated again, in a cycle that scientists believe may last 4,000 years or even tens of thousands of years before they escape to begin the eight-minute journey to your retina.
To a layperson, that the sunlight now illuminating the world may be composed of "old" photons—energy born as long as 100,000 years ago—makes little sense, but scientists understand why it happens. They've figured out the physics that underlies the phenomenon. Just like they understand what's behind another curious fact: The sun's equator spins faster than its polar regions. Were you able to stand on the equator, you'd complete a revolution in about 25 days, versus 35 days near the pole. And they understand why the sun generates sound waves that cause the surface to oscillate in three- and five-minute cycles, as if it has a pulse. Odd, yes, but explicable.
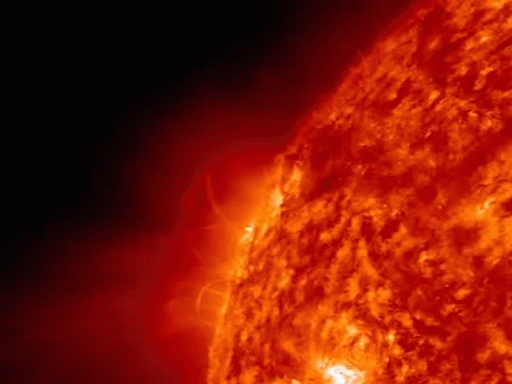
Image caption: From time to time, a solar flare or ejection will produce an explosive burst of billions of tons of plasma called a coronal mass ejection
As you move away from the sun, though, things get weird in ways that cannot be explained. The sun does not end at its visible surface. Consider the corona, the glowing plasma of electrons and the nuclei of hydrogen, helium, and heavier elements such as iron that becomes visible during a total eclipse. What to the eye is the sun's surface, which scientists call the photosphere, has a maximum temperature of around 10,000 degrees Fahrenheit. The corona's temperature can range from 1.7 million degrees to 10 times that. What? Imagine warming yourself on a winter's day by a bonfire, then walking away and instead of the air getting colder it gets hotter, a lot hotter. How can that be? What accounts for the corona's being so much hotter than the sun's photosphere? The energy comes from the sun's powerful magnetic field, but how or where is it dissipated to the corona? Nobody knows.
The super-hot corona energizes a stream of charged particles that constantly moves out and away from the sun. This is the solar wind, and as it moves out into space it accelerates to an average of about 250 miles per second. Why? How? Heliophysicist Nicola Fox explains: "If you just say, '[As an experimental model] we're going to dump in enough energy to make this acceleration start, don't worry where it comes from, just put x amount of energy here to cause an acceleration that's equal to what we see in the solar wind,' if you just do that, [the model says that] over time the solar wind should slow down dramatically. But it doesn't. So the explanation has to be more than just dumping a big piece of energy in there. There has to be something that is keeping the solar wind rolling at that speed." For the solar wind to flow continuously, there has to be a continuous supply of energy.
Again, though various scientists have hypotheses, nobody knows. Pondering these questions is what Fox does for a living. She is project scientist for the Parker Solar Probe, a spacecraft built by the Johns Hopkins Applied Physics Laboratory that is now on its way to the sun. If all goes well, during the next seven years the spacecraft—mission scientists tend to call it PSP or simply Probe—will orbit the sun 24 times and come within 4 million miles at its nearest approach. That may not sound all that close, but it's seven times closer than any previous mission, so close it will observe the solar wind when the plasma stream is still subsonic, so close that it will fly through the sun's atmosphere and encounter temperatures as high as 2,500 degrees. A unique heat shield, designed by APL and the Whiting School of Engineering, will keep the scientific instruments in its shadow working at around 85 degrees. The spacecraft's first close swing around the sun should take place in early November, and eager scientists should begin receiving data sometime in early 2019. They cannot wait. "It's by far the most exciting mission I can imagine," Fox says.
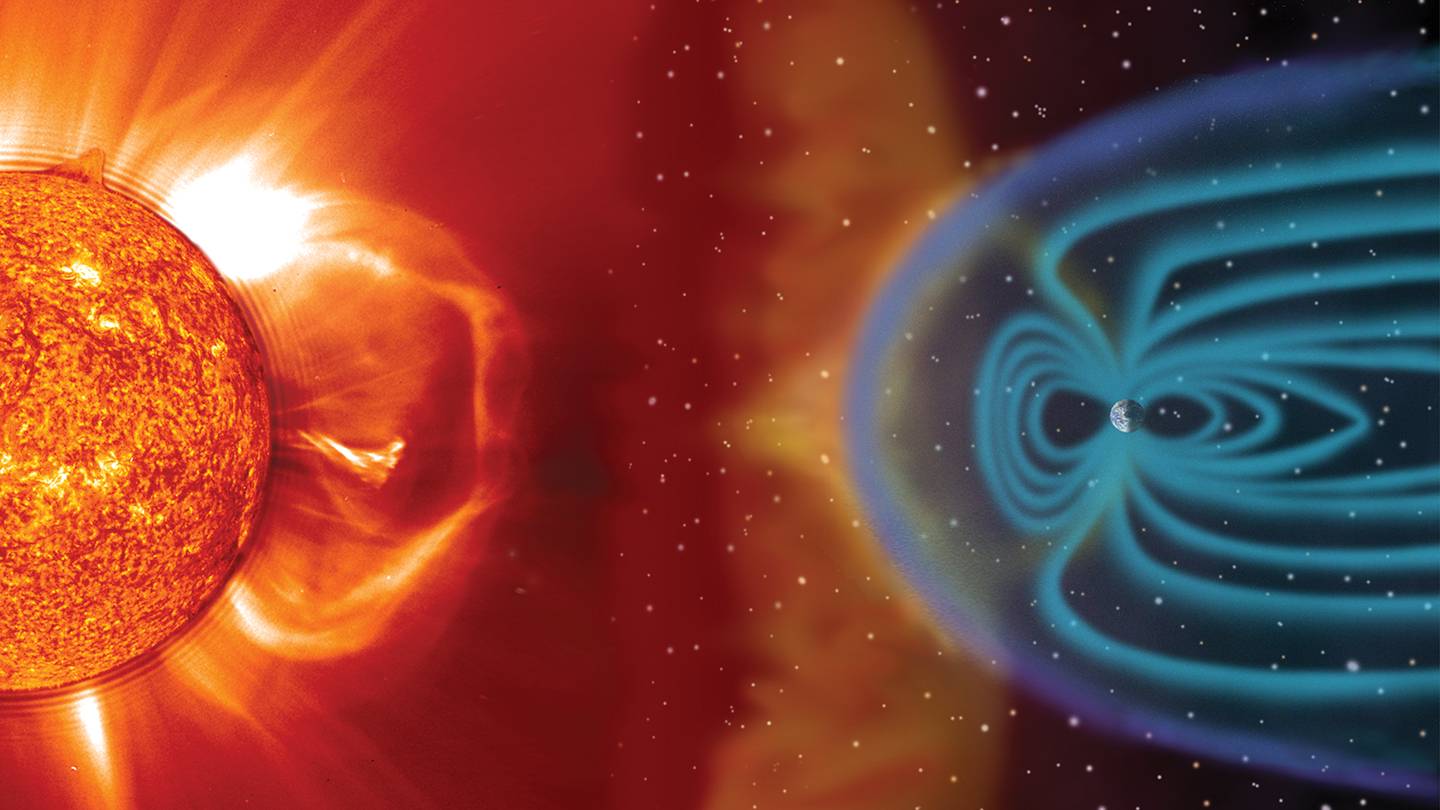
Image caption: Coronal mass ejection blast and subsequent impact on Earth
Image credit: SOHO/IASCO/EIT (ESA & NASA)
Scientists expect the data from the probe's instruments will enhance their understanding of space weather, the generic term for the ever-changing conditions in space around Earth. Space may seem empty and static, but it's not. The driver of our local solar system space weather is the solar wind, which appears insubstantial—an astronaut floating outside a space station can't see it or feel it or detect it without instruments—but it can have a profound effect on Earth. Just the wind's normal stream of charged particles and embedded magnetic fields would render Earth uninhabitable were it not for our planet's magnetic field, which deflects most of it like a wind shelter. The force of the solar wind actually deforms Earth's magnetic field, flattening it on the sun side and stretching it out behind the planet on the night side in a shape that resembles an onion.
From time to time, a solar flare or ejection of a ropelike coronal magnetic field structure will occur, producing an explosive burst of billions of tons of plasma called a coronal mass ejection. A CME carries its own intense magnetic field and travels at extraordinary speed through space; at its front edge it drives a shock wave through the solar wind. When this shock wave and the CME smash into Earth's magnetic field, they cause a geomagnetic storm. The mildest effect is very pretty: the dancing lights of an aurora. But there can be more serious consequences, ranging from an annoying disruption of the GPS navigation system to forcing the rerouting of airliners away from polar regions to ruining an orbiting satellite to a massive failure of the terrestrial power grid. On March 9, 1989, the sun flung off a CME that, four days later, crashed the Hydro-Québec power network; 6 million Canadians were without power for nine hours. Scott McIntosh, director of the High Altitude Observatory in Boulder, Colorado, has imagined a solar storm strong enough to generate a surge in electric current that takes out transformers up and down the Washington-Philadelphia-New York corridor. McIntosh points out that those cities don't have spare transformers just sitting in a warehouse in case of emergency, waiting to be plugged in. The grid could be down for an extended period of time, shutting down water systems, sewage treatment, transportation, communications, hospitals, financial markets, and on and on. McIntosh succinctly describes the potential damage as "trillions of dollars of infrastructure up in smoke." Not understanding space weather makes it much harder to forecast or prepare for the next big storm.
Scientists have been monitoring the sun from orbiting platforms since 1962, when NASA launched the first of a series of prosaically named Orbiting Solar Observatories. SOHO, the Solar and Heliospheric Observatory, was launched in 1995; the goal was to gather data for two years, but SOHO continues to function nearly 23 years on. The Ulysses spacecraft and the Advanced Composition Explorer have gathered data on the solar wind. The twin Solar Terrestrial Relations Observatory spacecraft beams stereoscopic images of the sun and various solar phenomena back to Earth. Over the years there have been the Solar Dynamics Observatory and the Transition Region and Coronal Explorer. All have collected valuable data and contributed much to understanding how the sun works, but all have the same limitation—they're too far away to have any hope of answering big questions about the corona and solar wind. Parker Solar Probe will fly close enough to the source to collect data of unprecedented granularity, before it has evolved over the several days of its journey to Earth. One scientist uses this analogy: Before now, studying the solar wind has been like observing a blizzard from 10 miles away; now we're going to drive right through it, with snow and ice pellets hitting the windshield. Fox calls it going in "where the magic is happening."
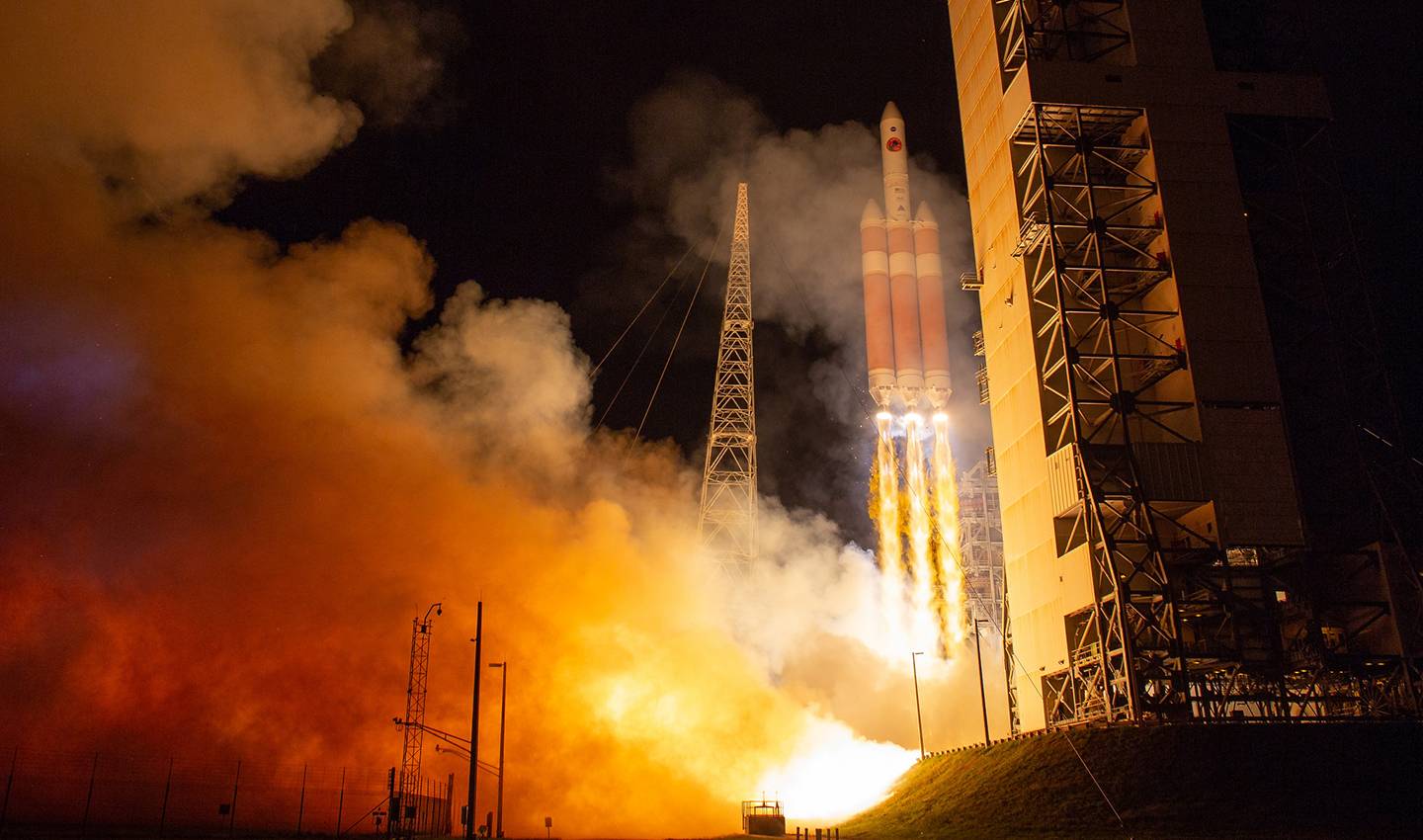
Image caption: The Parker Solar Probe launched from Cape Canaveral Air Force Station in Florida aboard a Delta IV Heavy rocket at 3:31 a.m. on the morning of Aug. 12.
Image credit: Bill Ingalls / NASA
Four sets of instruments on the probe will take measurements and capture images. Marco Velli, a professor of space physics at UCLA and one of the mission's five principal investigators, says, "These are the fundamental questions that Probe wants to address: Why does the sun have a corona at all, and what is the basic phenomenon producing coronal heating and the acceleration of the different types of solar wind? How do magnetic fields of the outer solar atmosphere define the basic structure of the solar wind? And third, how are energetic particles accelerated during massive solar events? My analogy is you can't understand a supersonic engine by studying the exhaust. You have to go into the engine. It's not a simple thing. There's really some basic physics that we have to learn."
Parker Solar Probe is named after Eugene N. Parker, the solar astrophysicist, now in his 90s, who first theorized the existence of a supersonic solar wind. Parker believes that the massive heating of the corona results from tiny events that he calls nanoflares. Within the sun, the energy generated by fusion slowly radiates from the core until, about two-thirds of the way to the surface, it enters a layer of churning plasma called the convective zone. The constant churning generates intense magnetic fields that structure the corona, and these fields are in constant flux as well, which causes the field lines to snap and reconnect. Each time this magnetic reconnection happens, there's a release of energy—a nanoflare. At a 2015 conference, James Klimchuk, a solar scientist at NASA's Goddard Space Flight Center, said, "The explosions are called nanoflares because they have one-billionth the energy of a regular [solar] flare. Despite being tiny by solar standards, each packs the wallop of a hydrogen bomb. Millions of them are going off every second across the sun, and collectively they heat the corona."
That is, they heat the corona if Parker, Klimchuk, and others are right. There are competing hypotheses, however. No part of the sun is ever static. It constantly seethes, vibrates, erupts, and fluctuates, and its magnetic field lines vibrate as well, creating all manner of waves. One such twisting oscillation is called an Alfvén wave; the mere presence of these waves was hypothetical until they were observed in the corona in 2007. Some scientists believe Alfvén waves carry the energy that heats the corona. Others suggest that the sun's photosphere generates magnetic waves that carry energy through the sun's atmosphere until they turn into shock waves that dissipate energy as heat in the corona; the energy is still ultimately magnetic energy, though it may turn into acoustic energy. One more idea: Vortices of whirling plasma and tangled magnetic field lines—massive solar tornadoes, of a sort—may transport energy to the corona. In 2012, an international team observed 14 of these tornadoes occurring within an hour and estimated that at any one time there are 11,000 of them whirling about the sun. These ideas are not all mutually exclusive. Nicholeen Viall, a research astrophysicist at Goddard who has worked on data from the Solar Dynamics Observatory, notes that Alfvén waves can induce magnetic reconnection, an energy source, and reconnection can, in turn, induce waves.
Russell Howard, a researcher with the U.S. Naval Research Laboratory, is principal investigator for the wide-field imager, PSP's equivalent of a camera. He says, "The problem is there are too many theories. Maybe there's more than one process going on. Certainly there are nanoflares on the sun, no question about that, but are they the source of the heating?"
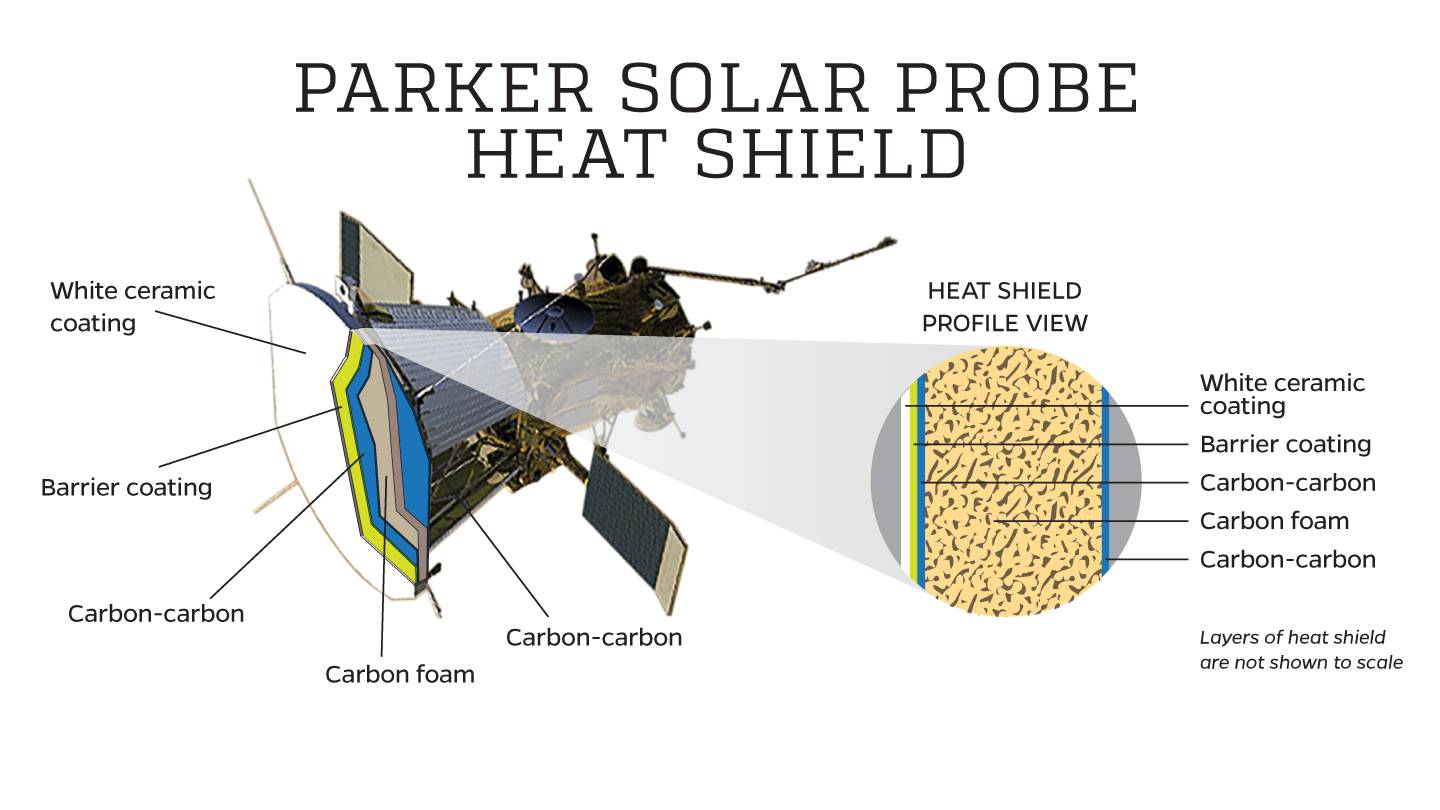
Image credit: Greg Stanley / Office of Communications
Streaming away from the corona, the solar wind is not a smooth and constant flow of protons and electrons, like a placid river, but an ever-changing phenomenon with a complex, fluctuating structure that's poorly understood. For example, there's actually a fast solar wind that covers 500 miles per second, and a slow solar wind that travels about half that speed. The two originate at different parts of the sun and vary widely in temperature and density. The closer to the sun, the more chaotic the wind's structure. Explosions that heat the corona may give rise to massive jets that merge as they expand outward; seen from afar, they resemble a continuous stream, but up close reveal an intermittent nature. "By the time the solar wind gets to Mercury, a lot [of the complexity] is sort of averaged out," Fox says. By flying so close to the sun, Parker Solar Probe will, in McIntosh's words, "put a big thermometer into the mess."
Levels of solar radiation, magnetic activity, sunspots, and CMEs follow a cycle of roughly 11 years. The sun's magnetic field reverses polarity on the same cycle. The current cycle will conclude around 2020, which has some of the PSP scientists excited. "It sounds a wee bit wacky, but what we've identified from looking at 140 years of solar cycle data are these events that happen every decade or so," McIntosh says. "We've dubbed them 'terminators' because the last two have roughly coincided with the appearance of a James Cameron Terminator movie. A terminator is the death of the last solar cycle. You'd think for a star, the end of a solar cycle would be a gradual thing, but it's really not. It takes place on a time scale of less than a solar rotation, so less than 27 days. We're predicting a fairly large event will take place probably in early 2020. There may even be an interplanetary shock associated with it." The spacecraft will be orbiting the sun if and when this occurs. "We're going to be in prime position. We've never had such a beautiful set of sensors to see an event like this."
McIntosh isn't the only one hoping for a ringside seat to a major solar eruption. "It would be very cool if we could fly through one of these giant space weather events, like one of these coronal mass ejections," Viall says. "I think there's a good chance that's going to happen. But to use a weather analogy, even if we missed a hurricane, there's still weather, and there's the solar wind happening all the time and no way we're going to miss that. We're going to get to see this plasma right after it's been energized." Echoing McIntosh, she adds, "We're going to stick a thermometer in right after it's been heated."
Viall, McIntosh, Velli, and Fox all can sound giddy at the science that Parker Solar Probe should make possible. "I've been working on this stuff for 15 years now," Velli says. "I'm kind of having trouble sleeping already." Thinking about how close to the sun the spacecraft will fly, Howard says, "It's just flabbergasting. It's just mind-boggling to me."
Nicola Fox can't wait. "You understand what happens in our star, it has applications for other stars. It's all particle acceleration. We have particle acceleration in the radiation belts, we have particle acceleration that powers the aurora, there's particle acceleration that causes the Crab Nebula to glow in X-rays. It's the same phenomenon, but it happens throughout the universe. There are going to be stars that are like ours that power interstellar weather, so they are going to have similar flares. We know that other stars have flares, we've seen that with Hubble. Would they probably have coronal mass ejections? Do they have coronas that are hotter than the surface? You know, why do they have that? Probably the same reason that we have it at our star. When you start thinking about exoplanets, what physics is going on at their stars, and how does that make for a habitable planet? I think that's why we've all got so excited about this mission: It's answering such fundamental questions that have applications throughout astrophysics. I mean, it's really amazing. Planetary physics—you know, a lot of the planets are driven by the solar wind. You understand how the solar wind works, you understand how those planets respond much better. It just transcends all the disciplines of science."
She pauses and smiles at her own exuberance. "Can I get an amen?"
Posted in Science+Technology