The hills of science are littered with wreckage, the remnants of failed attempts to touch the heavens of knowledge. The case of Otto Lilienthal explains this all too clearly. A late 19th-century German inventor, Lilienthal wowed his compatriots with newfangled flying machines. He buckled himself into a developing series of contraptions, jumped off hills outside Berlin, and soared around them. As he and his brother, Gustav, honed their glider technology, Lilienthal climbed to nearly 1,200 feet. In the process, he converted himself from oddball to visionary, drawing ever-larger crowds to his experiments. He made human flight seem possible.
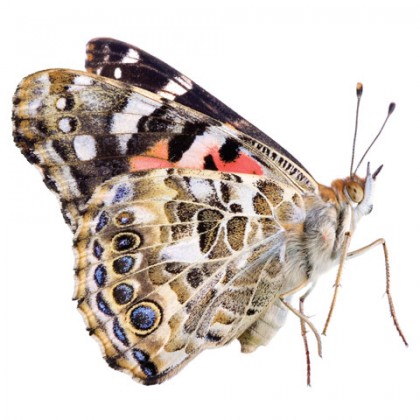
In his attempt to perfect his gliders, Lilienthal took clues from nature, especially the flight dynamics and wing conformations of the white stork. In 1889, he published Birdflight as the Basis of Aviation, in which he speculated that aircraft that imitated the flapping wings of birds could generate enough power to stay aloft. He incorporated into his gliders some of his theories on shifting one's weight to maintain flight, but he wouldn't have time to work out all of his ideas. In August 1896, after one last successful voyage, Lilienthal's glider lost contact with the drafts that had suspended him in midair some 2,000 times before. Lilienthal plunged 56 feet back to earth, breaking his neck. He died a day later, using his last breath to tell Gustav that in science, "sacrifices must be made."
If it could, Lilienthal's legacy would comfort him—Berlin's airport is named after him, and his work inspired the Wright brothers in their much less tragic quest for sustained human flight. And, despite Lilienthal's sacrifice, scientists today happily follow in his footsteps. They consider him the father of biomimetics, the study of the unique characteristics of animals, undertaken to create something of value from what we learn.
Part biology and part engineering, biomimetics and its nature-inspired spinoffs (such as the engineering-centered field of biomorphics) mimic individual species' evolutionary talents, then use the underlying principles to devise new medical treatments, craft more lifelike human replacement parts, and build cleverer robots. The fields regularly yield improvements in technology. For example, scientists who observed the gecko's ability to cling to walls have developed a sticky substance similar to what the gecko uses to defy gravity. Others have investigated the self-cleaning surface of sharks to develop boat hulls that resist contamination or staining by oil and other nuisances.
Some animals have such strange superpowers that they invite investigation just to see how they do what they do. Take the hawk moth. It contains an unfolding proboscis that is one and a half times its body length. It uses significant pumping power to pull nectar from plants into its belly. Could we make a moth-inspired mechanical pump that is as powerful? Other examples abound. Might the planarian worm's endless ability to regenerate yield clues as to how to slow cells' aging? Is it possible that the bacteria-killing peptides secreted by the skin of certain Australian frogs could provide humans with hints about protection from antibiotic-resistant infections?
"What's exciting about this kind of research is that bio-inspiration is all around us," says Rajat Mittal, a professor of mechanical engineering at the Whiting School of Engineering. "You never know where you'll find a novel solution." Mittal became nature motivated 15 years ago while watching a NASA presentation at the University of Florida, where he served as an assistant professor. A NASA scientist, echoing Lilienthal, was talking about creating an aircraft that could change the shape of its wings in midflight, as some birds and insects can. "I had never in my life thought we could learn anything from birds about aircraft design," Mittal says. From that moment on, he began to search in earnest for nature-centric solutions to mechanical problems, making it one of the focal points of his career.
Mittal is hardly alone. Since the turn of the century, bio-inspired research has grown by leaps and bounds, he says, including at Johns Hopkins University. Scientists at the Applied Physics Laboratory have studied hypersensitive dog noses to learn how they might develop a mechanical smell detector that could be used in airports or in contaminated regions too dangerous to risk search-and-rescue dogs. Engineers at the Whiting School who study neuromorphics—the science of creating networks of animal-like "nerves" in computerized robots and prostheses—researched lamprey eels to figure out how to electrically mimic the eel's ability to regenerate a severed spinal cord.
There are several ongoing experiments at Johns Hopkins that seek to unlock the secrets of animals. Here's a handful of the critters researchers are finding useful.
Squirrels
Admit a very ill human being to a hospital, put him in the intensive care unit, tend to him while he is comatose, and watch him (hopefully) recover. He still won't be completely healthy. Much of his muscle mass will have disappeared, so he'll weigh a good bit less and have difficulty moving. Such wasting is a challenge for patients who may have already been too weak to move around, especially the elderly. Four in 10 people over the age of 80 suffer from sarcopenia, an irreversible withering of the muscles. For younger people who have degenerative muscle diseases, such as muscular dystrophy, the search for a medical answer to wasting is a matter of life and death.
As part of that search, scientists have looked to certain mammals that sleep for seasons at a time but somehow manage to maintain their dexterity, strength, and muscle mass. One researcher, Ronald Cohn, an associate professor of genetics, neurology, and pediatrics in the School of Medicine, has spent much of the past five years examining squirrels for clues.
"When I was a boy of 10 or 11 years old, I was fascinated by bears and wondered how they could do what they do after hibernation," says Cohn. "But working with bears would be too unwieldy, so I landed on squirrels." Bears also shiver as they hibernate, perhaps enough to keep their muscles toned while they sleep. On the other hand, hibernating ground squirrels, which are native to the American Midwest and the Arctic, don't. They also don't eat, drink, or move for four to six of the coldest months of the year. Yet when they wake up, they run around without so much as a simple quad stretch. How?
To find out, Cohn keeps squirrels in automated hibernation for months at a time in his lab, during which, and afterward, lab workers perform chemical analysis and genetic testing on them. "We're looking to see if there is a master regulator, perhaps some kind of hormone or molecule, that has a domino effect on the molecular pathways in their muscles," Cohn explains. Finding such a chemical or chemicals would give researchers some idea of what's keeping their muscles toned, and what kind of drugs could be developed that could keep humans from wasting.
The problem is they haven't found a regulator yet. Cohn has learned, however, that the squirrel's entire body, including its organs and brain, goes through sweeping changes that likely begin even before it tucks itself in for its winter sleep. He just doesn't know how exactly it happens. "We haven't found patterns yet, or any answers as to why or how they go through these multiple changes," Cohn says.
But that doesn't mean the experiments have failed. As is the case with most basic science research, small discoveries add up. So far, Cohn has uncovered what he calls "a molecular signature of muscle endurance"—a number of proteins that signal how muscle mass is maintained. The way squirrels use those proteins is unique. "In essence, they're running a sprint and a marathon at the same time," Cohn says. "This is how they retain their muscle mass—even as they're hibernating."
Such clues may serve as the building blocks to cures for degenerative muscle diseases, Cohn adds. "The squirrel has figured out what science hasn't—yet. We've tried to study wasting for decades. Now, we've developed a good model to help us catch up with what squirrels innately understand."
Alligator Gars
For every fluffy, adorable creature like the squirrel that possesses an odd talent, there are several animals of seemingly less, er, aesthetic and anthropomorphic value that nonetheless prove useful to biomimetics and biomorphics. Consider the elusive alligator gar, a fish that swims near the surface of the lower Mississippi River and other freshwater rivers in the South and in Mexico. Capable of growing up to 10 feet long, the solitary gar is known for its jagged, alligator-like teeth and ungainly snout.
It may not make for a nice stuffed animal that ends up in a crib, but the alligator gar has several things going for it. It can live as long as 70 years and its scales are extremely hard (something that may factor into its longevity). That tough exterior has piqued the interest of the U.S. military, which regularly enlists the help of Johns Hopkins engineers. For the past several years, Whiting School researchers have sought insight from other waterborne creatures, including the abalone and its durable shell, in a search for clues as to how to build better armor for military cars, tanks, and infantry soldiers.
"What we're looking for are substances that are strong enough that they rarely break, are tough so that they maintain some integrity when they do break, and are of low weight," says K.T. Ramesh, a professor of mechanical engineering at the Whiting School. "The advantage in looking to nature for useful substances is that nature has developed a very wide range of possible microstructures over time, and selected the very successful few of them. Some have evolved interlocking hierarchical microstructures that make these surfaces tough." Evolution provides a valuable lesson for nature's mimics, he adds. "Nature does the opposite of what engineers usually do. We look for ways to build things simple and fast."
As an abalone shell develops, it grows different structures at the millimeter level than it does at the microscale and nanoscale. One level may be made up of a hardened material that makes the shell or scale strong, while a softer layer (usually a polymer) interlocks with it to make it tougher. Those tiers link together with other layers of tough stuff.
The alligator gar scale is similar. Recently, Ramesh and his lab mates began to look at the fish's interlocking ganoid scales, which are made up of enamel over a base, covered by a layer of ganoin, a shimmery hard material that makes up the scale's surface. The scales, shaped like diamonds, are prized for their durability—so much so that Native Americans used them to make arrowheads and jewelry. Gar scales and some seashell varieties, because they are complexly grown over time, are ripe for applications made by engineers. "When biomaterials are hit by something, the microstructure forces the cracks to take these long, convoluted pathways. Because damage to these pathways doesn't compromise the system involved, they retain some toughness," says Ramesh.
Over the coming years, Johns Hopkins engineers hope to investigate how individual scales hold up during testing. ("We're interested in the scale itself, not the gar scales' interlocking features," Ramesh explains.) They'll subject scales to repeated hits from "microscale projectiles," bits of material shot at high velocity. To document how the scales react to the barrage, the results will be recorded by a high-speed camera system. Researchers will measure the material's strength, create a theory to explain and quantify it, and then see if they can develop a similar material via research engineering—the art of modeling something natural into something that isn't. The resulting product could then be used to protect troops and equipment during battle.
Besides offering the benefit of having a model to study, bio-inspired research contains another advantage: It makes it easier to explain the work at hand to others, Ramesh says. "People—funders, students—can connect to a biological analog. Once the intuitive connection is made, it's much easier to engage people's intellects."
Ramesh's lab's approach indeed has its appeal. In April, it won a $90 million grant from the U.S. Army to lead a consortium of private industries and universities that will investigate the basic science behind materials, like a soldier's armor, that are subjected to tremendous impacts.
Songbirds
How do members of species cooperate? Are they programmed to dance or sing in tandem? Or do they learn how to work together?
To find answers, Eric Fortune, an associate professor of psychological and brain sciences in the Krieger School of Arts and Sciences, treks regularly to Ecuador, where he studies how male and female members of the species Pheugopedius euophrys—the plain-tailed wren—coordinate the territorial duets they sing on the side of an Andean volcano. In a study published in Science last December, Fortune reported that by analyzing the wrens' shared song and measuring their brain activity, he and his team discovered that the birds hear acoustic cues as they trade notes. They actually change sequences as they cooperate. "The wrens segregate those cues in a very discrete way in time," Fortune says. "Pairs of birds can sing the duets even when they can't see each other."
Even though they sing alone as well, their brains act differently—cooperatively—when they croon in pairs, evidence that they learn exactly how to do it. "We had expected the brains of each wren to respond most strongly to its own part, but surprisingly we found that neurons respond most strongly when the pair is singing the duet," says Fortune. "Each bird has a memory of the complete duet, which a single bird cannot produce alone."
Fortune's work has implications not just for birds but other species, including humans who dance in pairs, reading cues and coordinating movements in time as they tango. "When animals interact, it's incredibly complex," he says.
The study's first application may not be in the animal kingdom, however. Understanding how brains work together is important for developing machines that can mimic behavior in "animal time," as opposed to the regularity of the rhythms robots are usually programmed with. Fortune's findings allow scientists who work with robots to imbue them with the relatively imprecise time that people use when dealing with one another. Fortune and colleagues are using wren data to develop prostheses that respond more like human arms would, such as by recognizing when and how to shake hands, and how to work in tandem. "If you shake hands with a child, they don't always get what it's about—you have to instruct them what to do. It's the same with robotics. A prosthetic arm would need to have a memory of shaking a hand in order to cooperate and shake your hand in real human time," Fortune explains, adding that a "noisier, messier" timing mechanism could now be developed, based in part on his research.
As a biologist, Fortune finds the ever-present similarities between species fascinating. But because each has its own behaviors and physicality, science has a chance to learn something new and potentially valuable from every species. "Animals and humans are exactly the same and completely different at the same time," he says. "We may share 99 percent of our genetic material with chimps, which means that we're almost identical, but the devil is in the details—that 1 percent represents a large number of differences, an amazingly complex set of issues. We evolved from the same point, but the differences are telling."
Cockroaches
It might seem absurd that the vaunted National Science Foundation would grant millions of dollars to researchers investigating the utility of Periplaneta americana, the humble American cockroach. But it has, and for several years now, and there's a very good reason why: The cockroach's ability to instantaneously make 25 turns per second while following along a darkened wall is the result of a highly developed and calibrated system—one that could prove useful in making robots that travel blindly into dangerous areas during military operations and search-and-rescue missions.
Robot eyes don't navigate well without light, something that cockroaches, outfitted with a specialized antenna that measures how far they are from surfaces, excel at. The antenna does this using a sense of touch that complements its extraordinary design. The insect can run 20 body lengths per second in complete darkness without mishap, thanks to the 200 hairy, sensitized segments packed into the antenna's 5 centimeters. "The hairs on the antenna provide a steady flow of information on how far a cockroach is from the wall," says Noah Cowan, an associate professor of mechanical engineering at the Whiting School. With the help of about $100,000 in grant money, he has been researching the insect along with colleagues at Johns Hopkins and the University of California, Berkeley, for the past decade. "They allow the cockroach to gather and process very detailed information incredibly rapidly. They also serve the mechanical purpose of orienting the antenna to points where it is most useful," he adds.
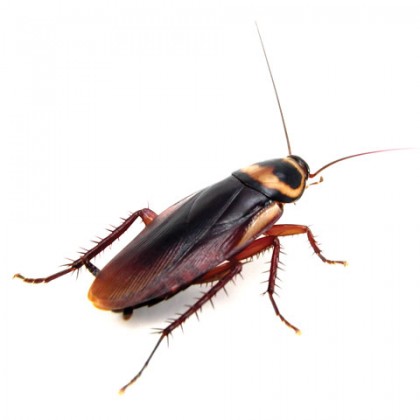
Image credit: istockphoto.com
As with many bio-inspired studies, Cowan's goal isn't to imitate nature completely. The cockroach's unique ability to situate its antenna in a certain way may be of interest to science but may or may not be relevant to engineering. "When you're translating biology into engineering, the first trick is to understand," he says. "Once you understand the principles behind a natural system, you can copy the elements that are most relevant—not necessarily the entire system itself." What's more, it is not always obvious to engineers how to copy a design because, Cowan says, "we tend to think in a more modular way. But animals have evolved complex, integrated systems over time. Teasing out the function of a system can be challenging because it has had to work on many levels at once, such as feeding, navigation, and reproduction. We must understand how nature's design applies to the engineering task at hand, and strip away features that serve no engineering purpose."
To get at the cockroach's special sensory powers—what engineering finds useful about the insect—Cowan and his crew used a laser to burn off the antenna's hairs to see what would happen without them. As an example of just how sensitive the cockroaches are, Cowan says they can sense, via pressure waves, when a human walks into a room. "That's why you rarely know when you have cockroaches in your house," he says. "They've already been traveling one and a half meters per second before you've even turned on the light."
After gathering information on the insect's sensitivity, the team did what bio-inspired engineers regularly do—they developed mathematical models of the antenna and the insect's behavior. That data has been used to build an antenna-like sensor that feeds navigational data to the robot's controller. Cowan hopes the design will be useful in robots that will make their way through tight spaces, such as in buildings that have collapsed during earthquakes, to find people who are trapped.
Research on animals keeps him focused on his robotics problems, Cowan says. "I don't think I even know how to do basic engineering science. It's easier for me to sink my teeth into trying to understand how an animal works. That helps me generate hypotheses. Then, I get to work in the lab. We often end up discovering things we never expected."
Sunfish
The U.S. military also has an interest in using robots underwater to identify mines, perform search and rescue, and navigate safe routes through hostile bays, oceans, and rivers. Traditionally, it has relied on trained dolphins to sniff out danger. But the highly intelligent mammals cost a lot to train, can become overstressed, and are mortal. Submersible robots called autonomous underwater vehicles (AUVs) promise to be cheaper and more reliable. But they have their issues as well, including not being able to move as smoothly and nimbly through water as a fish (or a dolphin) does. The typical engineering solution for watercraft—a propeller—is great for moving a vehicle forward fast and straight. But it's horrible for anything else, especially evasive maneuvers.
The answer? To study fish and how they glide and dart through water, and then create something mechanical that is very similar to it. "When I look at a fish, I see a creature that has evolved over a very long time and developed a variety of sophisticated engineering systems," says Mittal, whose job it is to convert military grants into AUVs. He gets help from biologists who understand fish. "There's an elegance to it—maybe too much elegance for our purposes."
Mittal, his team, and outside collaborators have been entrusted to build a robotic pectoral fin for a small AUV. Studying the graceful movements of the pliable fins of the bluegill sunfish proved valuable to his research—and counterintuitive, he says. Building something sturdy, even impregnable, may seem like a worthy goal for an engineer, but that doesn't apply in this instance. "What we learn from fish, and even from butterflies, is that flexible structures are, in fact, good," says Mittal. "As engineers, we've been trained to make things as strong and invulnerable as we can. In nature, maybe because there's no steel around, or maybe because it's smarter than we are, we see all these flexible systems that impart great advantages over rigid structures. All of this is opposite to how engineers have been taught to think."
As with Cowan's work, engineers have found an easier way to achieve the same effects as nature. The fin of a bluegill sunfish has 14 fingerlike rays that help it move smoothly and quickly through the drink. But Mittal found, with the usual aid of advanced math and computer modeling, that he needed fewer fins to make the submersible robot travel in ways similar to it. "Our computer models showed us that only five of the rays are needed to produce thrust and efficiency similar to the fish fin," he says. "The other rays aren't there to increase thrust but for other purposes, such as defense."
Mittal, who has also conducted research on the systems of various insects, tends to see bio-inspired solutions wherever he looks. Recently, while watching a BBC television program, he noticed that bubbles come off the bodies of penguins as they shoot out of the water. "Those bubbles could have some huge implications for drag reduction," he says excitedly, adding that he and another professor hope to study the birds. "Is the down of penguins covered with air, which would help them move faster through the water? It's an amazing opportunity to learn whether that's true and what it might mean."
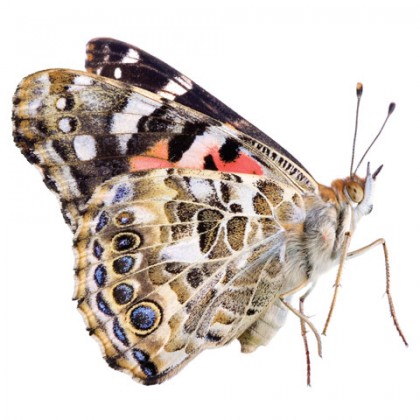
Image credit: istockphoto.com
Butterflies
Whiting School researchers don't limit their designing skills to robots that roam the land and sea. Aided in part by more military grants, they also investigate ways to fill the air with flying machines that can perform reconnaissance, rescue, and other military operations, particularly in dense, urban neighborhoods. In order to fly under the radar of various surveillance systems, the flying machines need to be small, very mobile, and easily directed by human controllers. The bug-sized robots also need to perform aerial maneuvers worthy of some of nature's most accomplished fliers. It makes sense, then, for engineers to learn as much as they can from butterflies, which are easier to manage during experiments than birds, and to see if the results can be applied to the development of so-called micro aerial vehicles (MAVs).
For the past two years, Tiras Lin, a 21-year-old junior mechanical engineering major from San Rafael, California, has investigated the motion of butterfly wings. The study was suggested to Lin by Mittal, his adviser on the project. Lin concentrated on the painted lady butterfly, a globally ubiquitous species that flaps its wings 25 times per second. Central to his study was Mittal's suggestion that engineers have too often underestimated the role a concept called "moment of inertia" plays during flight, especially when the insect is manuvering.
To describe the phenomenon, Lin draws a comparison to an ice skater performing a spin. "Just as the skater redistributes his mass by drawing his arms inward or outward to rotate faster or slower, butterflies modify their moment of inertia by reconfiguring their wings in flight," he says. Using pictures taken by three high-speed cameras of painted ladies inside an aquarium tank (at a rate up to 3,000 images per second), Lin discovered that flapping wings have a significant effect on the insect's moment of inertia—even though the wings themselves make up only about 10 percent of the insect's total mass. In other words, a low-weight part of the butterfly's body can have a disproportionately large effect on how it keeps itself aloft and hyper-maneuverable.
Understanding the role of the moment of inertia more clearly has major implications for designers of flying robots, Mittal says. "It's always been an idea that was swept under the rug. Butterflies turn 90 degrees with two wing flaps, which is incredible. Imagine an aircraft that could do that." As always, he's enthusiastic about other avenues for study. "Flies get swatted, yet can gather themselves and fly away, and we see wind gusts that blow flying insects around all the time, yet their flight is so robust in the face of all these perturbations. How do they do it?"
Posted in Health, Science+Technology
Tagged noah cowan, robotics, applied physics laboratory, genetics, ronald cohn, rajat mittal, biology, k.t. ramesh, eric fortune