Humans have long had a love-hate relationship with bacteria. While there are bacterial strains that humans cannot live without, such as those that help us digest our food and bolster our immune systems, there are many that we cannot live with, literally. Deadly diseases caused by pathogenic bacteria, such as cholera, syphilis, anthrax, leprosy, tuberculosis, tetanus, and bubonic plague, have claimed the lives of millions throughout human history.
Although our ability to treat and prevent the spread of such diseases has improved drastically over the past century, bacterial infections remain among the leading causes of death globally. Scientists estimate that these infections accounted for more than 1 in 8 deaths in 2019, making them the second leading cause of death that year. Our liberal use of antibiotics is also increasing the number of antibiotic-resistant strains of bacteria that doctors can do little to treat.
Bacteria are not something we can afford to ignore. Put simply, we need to better learn how to kill the bacteria that harm us and nurture the bacteria that help us. The key to doing that lies in answering a question that has been stumping scientists for centuries: How do bacteria get their power?
Princeton University molecular biologist Bonnie Lynn Bassler, A&S '90 (PhD), has spent decades leading trailblazing studies on how single-cell microscopic organisms can impact our health in such a profound way. And now she has an answer:
"They do it by talking, counting, and carrying out tasks in groups," she says.
Bassler was among the first to discover that bacteria communicate by exchanging molecules, a process known as quorum sensing, and she has forged a career out of eavesdropping on bacterial conversations. Her pioneering work has earned her a MacArthur Fellowship, the American Society for Microbiology's Eli Lilly Investigator Award, the Wiley Prize in Biomedical Sciences, and a spot on the National Science Board, to which President Barack Obama nominated her. Bassler won the 2023 Canada Gairdner International Award, which recognizes major contributions to the treatment of disease (418 awards have been given since its founding in 1957, and of those awardees, 98 have gone on to receive a Nobel Prize.) Her TED Talks have been viewed by millions. In one, back in 2009, she reminded people just how many more bacterial cells they have in them than human cells. "I know you think of yourself as human beings," she said, addressing the crowd. "But I think of you as 90% to 99% bacterial." They keep us alive. But they can do terrible things, too.
She is now developing a technique that seeks to silence bad bacteria, thus rendering them harmless. If successful, this quorum quenching could not only turn the tide in our war against bacterial infections but also revolutionize agriculture, water treatment, and so much more.
Bacteria play a role in virtually every aspect of our lives. They are in the oxygen we breathe, 20% of which they produce. They are among Earth's oldest (we're talking billions of years) and most abundant organisms, but much remains to be learned about them. Scientists had no idea these seemingly simple organisms could communicate until 1970 when John Woodland Hastings, a professor at Harvard University, and his colleagues stumbled across evidence while studying a bioluminescent bacterium known as Vibrio fischeri that is found in many marine animals. Hastings and his team noticed that the bacteria wouldn't emit their blue-green glow unless they had lots of their brethren around them. Upon further inspection, Hastings discovered that the bacteria emitted a molecule—which would later become known as an autoinducer—that stimulated collective glowing but only when the bacteria had reached a particular population density. In other words, the bacteria wouldn't get the party started till all their friends showed up.
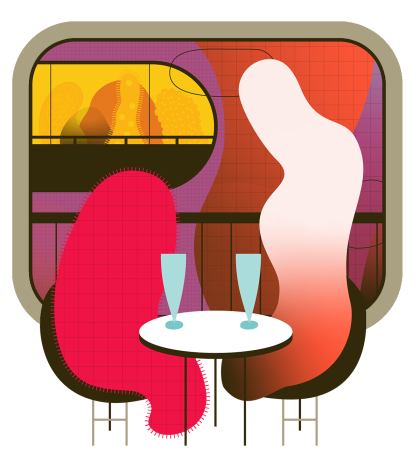
Image credit: Alex Eben Meyer
It would take a decade before another scientist, Mike Silverman, identified the gene linked with this mysterious signaling molecule, as well as the receptors that receive it. Silverman, then a researcher at the Agouron Institute in La Jolla, California, did so by chopping up a chromosome from V. fischeri, putting the pieces into petri dishes full of E. coli, and turning the lights off to see which E. coli colonies glowed.
"What was utterly remarkable about that experiment was not only did the E. coli make light, they only made light at high cell density, and they made that [signaling] molecule. [Silverman] got the molecule, the enzyme that made the molecule, the receptor for the molecule, and he got the light genes all on one piece of DNA. He figured it all out," Bassler says.
The communication circuit Silverman discovered explained how V. fischeri were able to coordinate their luminous displays. This mechanism explains how bobtail squid, one of the many marine animals that host colonies of V. fischeri, make use of the bacteria. When the sun goes down, they use the glowing V. fischeri stored in their light organ to help them blend in with the moonlit surface waters while they hunt. When the sun rises, these squid expel 95% of their V. fischeri and bury themselves beneath the sand to avoid being seen by predators. As they hide, the few bacteria that remain multiply, and by the time the sun sets again, the bacteria will be at a high enough density to trigger their gorgeous glow. Shortly after making his discovery, Silverman met Bassler at a conference where he was giving a talk on his work. The budding young scientist was just finishing her biochemistry doctorate at Johns Hopkins at the time.
Bassler says she got into science "accidentally." She grew up loving animals and nature and had anticipated a career as a vet. But not far into her studies at the UC Davis, she realized it was an utter mismatch. "Because I really like live animals, and I don't want to cut them open," she says. "I don't want to memorize their bones; I don't want to see them when they're sick. I didn't last very long." She changed her major to biochemistry.
Then, during her junior year of college, Bassler's mom died from colon cancer, a mere four months after she was diagnosed. Bassler became determined to help discover a cure and make major contributions to medicine. She applied for a volunteer position in a lab that was studying the disease but ended up being put to work on a project that involved bacteria. "It was truly just a fluke because when I started working with them, I just fell in love with bacteria," Bassler says. She found studying these tiny organisms to be the perfect blend of her interest in nature and her knack for solving puzzles. "It was just so fun. I never wanted to leave the bench." After graduating from Johns Hopkins with a doctorate in biochemistry, she began looking for a way to return to the bench. That's when she ran into Silverman. "After he gave this mesmerizing talk, I literally ran up to the podium and said, 'You have to let me be your postdoc,'" Bassler recalls.
Not long after Bassler and Silverman teamed up, they found that bacteria produce more than one type of autoinducer. It was clear that bacteria were saying more than, "Hey, I'm here." Around the same time, other scientists were finding autoinducers in pathogenic bacteria and theorizing that they play a role in the pathogen's virulence. The term "quorum sensing" was coined soon after, and Bassler founded a lab at Princeton dedicated to studying it. Then, in the late 1990s, Bassler discovered something that would forever change the way we think about bacteria.
She identified a new autoinducer, isolated the gene responsible for its production, and searched the bacterial genome library to see if any other bacterial species had the same gene.
"I was sitting in front of this clunky old computer with my colleague Mike Surette. We sent our gene off, and then every bacterium came back with that gene. There were only 40 bacterial genomes, but it was all the bacteria that had ever been sequenced. I remember sitting there getting goose pimples. I said, 'Oh, my God, they're talking to each other,'" Bassler says. Incredibly, bacteria of all kinds appeared to be speaking the same language. It was then that she realized that chemical communication was ubiquitous among bacteria. She discovered that all bacteria produce the same autoinducer, also known as the universal communication molecule or "autoinducer 2," allowing them to communicate across species.
Bassler next began to wonder whether bacteria had species-specific languages. She examined all known autoinducers and noticed an interesting pattern: They all shared the same base structure, but many had a unique addition for intraspecific (i.e., within one species) communication. Think of this chatter as "private, secret conversations," she says. A language only members of that species could understand.
Bacteria live in diverse environments alongside hundreds, sometimes thousands, of other species. Scientists estimate that each square centimeter of our skin contains 1 million bacteria from hundreds of distinct species. With species-specific autoinducers and autoinducer receptors, bacteria can communicate privately with their kin while swimming in a sea of other bacterial species. Having a secret language also allows them to identify who is kin and who isn't.
"The bacteria measure the ratios of these molecules, and then they change their behaviors based on whether they're surrounded by kin or they're surrounded by the enemy," Bassler says.
When surrounded by friends and family, bacteria will share resources and help one another. But when surrounded by competitors, they cease their sharing and may even coordinate an attack. "This should sound very familiar; it's what we do," Bassler says, referring to the fact that the cells in higher organisms, including those in our body, have the same capacity. "We proved that bacteria evolved that capacity billions of years ago. It's not special for eukaryotic cells. It also exists in the bacterial world."
Bassler and her team discovered that bacteria are, for all intents and purposes, multicellular beings. They are capable of communication within and across species, possess the ability to distinguish themselves from others, and can use these abilities to work together to accomplish their goals.
"Everybody thought bacteria were kind of stupid," Bassler says. "They thought that all the big mysteries in bacteria had been solved. But there were scientists who really believed that bacteria still had secrets to spill. It turned out they were right."
This revelation got Bassler thinking about how this power could be hijacked for the benefit of humankind. If you could make molecules that interfere with quorum sensing, she thought, you could make pathogenic bacteria avirulent—in other words, harmless.
If scientists could prevent quorum sensing by disrupting signaling, a process now known as quorum quenching, they could create new antibiotics that could save millions of lives, revolutionize agriculture by neutralizing bacterial pathogens that plague the plants and animals we farm, and prevent the growth of bacterial biofilms on medical devices, transportation infrastructure, and water systems. The possibilities of quorum quenching are seemingly endless.
Also see
Bassler's work "identified key molecular players and also outlined the pathways involved in [quorum-sensing] processes, paving the way for different therapeutic approaches to block bacterial communication and combat bacterial infections," says Kimberly Davis, an associate professor and microbiologist at the Johns Hopkins Bloomberg School of Public Health.
Davis believes quorum quenching "could be an exciting way of blocking bacterial behaviors that contribute to human disease," adding that it could prevent bacteria from causing host tissue damage in the first place, and, if used alongside antibiotic treatments, then eliminate the bad bacterial cells. Think of it like silence and erase.
"I'm slightly biased because I am brainwashed to love bacteria and quorum sensing," she says, "but there's an amazing amount of work to be done. And it's all brand new."
For the past two decades, Bassler and dozens of other scientists have been hard at work trying to make quorum quenching a reality. Bassler has already developed several synthetic quorum-quenching molecules that are essentially disguised antagonists that block the real autoinducer from interacting and turning on the quorum-sensing cascade.
When bacteria infect a host, they don't attack right away. They use their communication and counting abilities and wait until they are abundant enough to launch an attack that's likely to succeed. Bassler began testing her quorum-quenching molecules by introducing them into nematodes alongside pseudomonas, a pathogenic bacterium commonly found in health care facilities, whose quorum sensing they were designed to disrupt. In 2009, Bassler and her team had their first success, becoming the first lab to develop a synthetic quorum-quenching molecule that shut down virulence in an animal model.
The synthetic molecule used in this study isn't ready for use in humans yet, but Bassler and her colleagues have been investigating how to make it so. One of the ways they are doing this is by taking inspiration from nature. Other scientists recently discovered that some eukaryotic cells, including human cells, produce quorum-sensing-interfering molecules, not unlike Bassler's synthetic ones, that can positively and negatively influence bacterial quorum sensing.
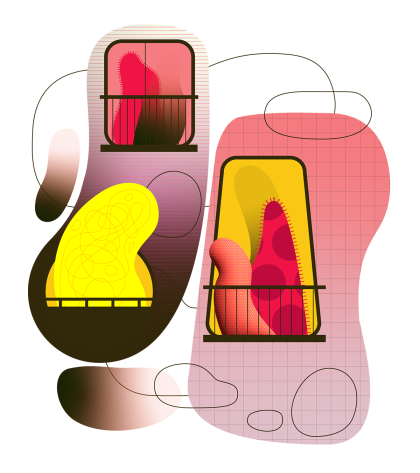
Image credit: Alex Eben Meyer
Bassler and her colleagues are also looking into ways to embed synthetic quorum-quenching molecules into materials, paving the way for the development of infection-resistant medical devices such as catheters or breathing tubes.
Bassler's work has the potential to turn the tide on our war against bacterial infection, and it may also aid in our fight against viral infection. She recently discovered that dozens of viruses respond to quorum sensing or other chemical signals from bacteria. "We've realized that viruses that infect bacteria eavesdrop on bacterial quorum sensing," she says. "We now know quorum sensing transcends all domains on Earth, from viruses to bacteria to eukaryotes."
When it comes to understanding bacteria and their ability to communicate, "we've barely scratched the surface," Bassler says. "If we want to make quorum sensing better or stop quorum sensing, we have to be able to do it outside of a lab, and we don't know how to do that because the field is young."
Over the past 30 years, Bassler has completely transformed our understanding of bacteria, their capabilities, and their profound influence on nature and medicine. She says that much like the bacteria she studies, her success can be credited to her ability to work with others. "I've always liked the people part of [science]," she says. "Ideas get tossed around, it's interactive, it's collaborative, and it's fun." She currently has her sights set on making quorum quenching a feasible and functional tool for future scientists. Although she has no plans to end her research career anytime soon, she believes it will be the next generation of scientists, such as the graduate students in her lab, who will bring this technology to its full potential. "I have the privilege of working with tomorrow's future leaders in science. They're creative, they're smart, they're wonderful people. It's what keeps me going."
Posted in Science+Technology