On top of the world, where the North American and European continents meet, the planet's largest waterfall swirls and tumbles over a dramatic tectonic rift. The Denmark Strait cataract is the greatest waterfall you've never seen, because it falls entirely under the surface of the ocean. The current of water, pushed north along the Gulf Stream and cooled by the frigid Arctic, suddenly drops 11,500 feet over a 60-mile-wide cliff between Greenland and Iceland. Because cold water sinks, this torrent descends into the deep ocean, often emerging a world away, near Antarctica. Churning waves in the Southern Ocean, driven by terrific winds, then force the water north once more, along the coasts of South and North America.
This meandering loop is known as the Atlantic meridional overturning circulation, or AMOC, a 10,000-mile-long conveyor belt of ocean currents that moves heat and water—and effectively our weather and seafood—around the globe. The loop is driven by forces such as the cold water descending in the Arctic, but as oceans warm and change, this loop could break at key points—which is bad news for the ocean's ability to continue storing heat and keeping our planet cool. Even slight changes to the ocean's temperature, density, and composition can have far-flung implications.
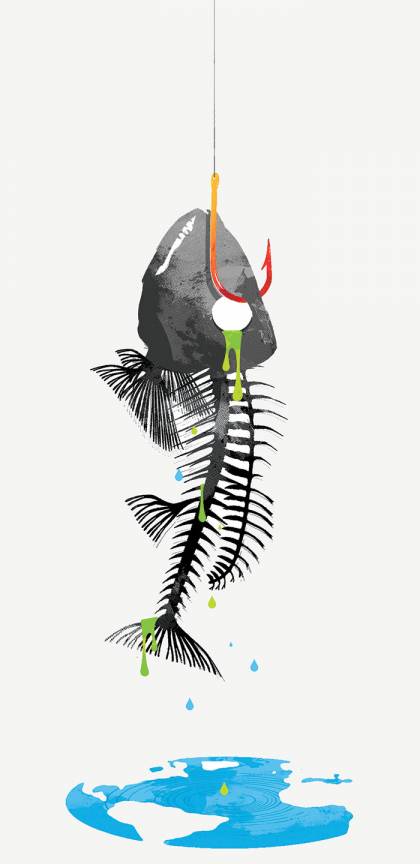
Image credit: Gary Neill
As you probably remember from physics class, warm water expands, taking up more room. As oceans absorb more thermal heat from human-caused emissions, the seas rise and flood shorelines, destroying the homes and communities of hundreds of millions of people. New Orleans could disappear in the next 80 years because of unchecked sea-level rise. Anand Gnanadesikan, chair of the Johns Hopkins Department of Earth and Planetary Sciences with a background in physical oceanography, says the most vulnerable city on the U.S. East Coast is Annapolis, Maryland, because the land around and under the Chesapeake Bay is sinking as a result of natural causes at the same time seas are rising because of climate change.
Climate-induced changes on oceans will also affect our weather, creating more ruinous hurricanes, debilitating snowstorms, scorching droughts, and apocalyptic wildfires. In 2012, Superstorm Sandy battered the Atlantic coastline from the Caribbean to Canada. It flooded the New York City subway and blanketed states like West Virginia in deep snow, causing power outages for weeks. All told, the storm caused an estimated $70 billion in damages. A storm like Sandy, which may have happened every hundred years or so before these dramatic ocean changes became visible a few decades ago, could flood New York every seven years by 2050 or so, Gnanadesikan says.
With the steady annual rise in greenhouse gas emissions—which trap heat in the atmosphere and warm the globe—humans are speeding up the destruction. The ocean surface warms as the air does, creating swaths of water that rise in height and feed into dangerous storms. Some of the changes wreaking havoc are likely to continue for a time even if emissions drop, since the effects of what happens now may not be felt for hundreds of years.
Our oceans, meanwhile, are working overtime to correct the balance. Oceans, which cover 71% of the planet and contain 97% of its water, hold as much heat in the top few feet of the surface as the entire atmosphere. About one-third of the carbon dioxide released into the atmosphere and 93% of the heat trapped by those emissions are sucked into oceans, which move that excess heat around the globe. If rainforests like the Amazon work as the planet's lungs by releasing oxygen and storing carbon dioxide, oceans are its heart and circulatory system, threading oxygen and nutrients through the entire system.
And oceans work on immense time scales, with thousand-year-old water still flowing through the deep ocean—perhaps containing remnants of the currents that carried the Vikings to North America. "The ocean is the memory of the Earth's climate system," Gnanadesikan says. Below these ancient currents lie rocks and sediment that tell us the stories of climates gone by—and offer a glimpse of the future if we continue warming the planet at rates not seen in millions of years.
Oceans do the planet an amazing favor. They absorb carbon dioxide from the atmosphere wherever air meets water, and winds cause waves and turbulence, giving this process a boost. But what happens if the balance shifts? Is it possible for oceans to stop absorbing heat and greenhouse gas emissions? And if that happens, will the world's ocean systems go (even more) haywire? These are exactly the questions researchers are trying to answer, looking everywhere from the Arctic and Antarctica to the Chesapeake Bay. What they know so far is deeply concerning: Our unrelenting emissions are stressing oceans out, and they might not be able to help us as much—or, in some troubling scenarios, at all—in the future.
It all goes back to that waterfall and ocean circulation, driven by the cool, dense water descending. "But if we have warmer temperatures, then the water doesn't get as cold," says Maya Gomes, an assistant professor in the Department of Earth and Planetary Sciences who studies the geochemistry of current and ancient oceans. "So that means that the ocean doesn't circulate as quickly." And if it's not circulating as quickly, then less of the ocean interacts with the atmosphere, which means less oxygen is pulled into those waters.
On top of all that, Gomes says, the water that's coming into the ocean—from rainfall and rivers—"will be warmer, and so it'll have less oxygen in the first place." Low oxygen conditions can create dead zones that harm marine life, like fish, and the people who rely on it. With nearly half the world depending on oceans for food, such changes make it harder to know when our next meal will be and where it will come from.
"The climate's already changing," says Darryn Waugh, a professor in the Department of Earth and Planetary Sciences who specializes in ocean circulation and dynamics, and the ocean's role in climate. "We're starting to see the impacts of that. And in the future, how bad it could be depends on how the oceans change."
Of all the places on the globe, the Arctic may be the hardest hit by climate change. It is ground zero of global warming, where you can look out your window and see the effects of a heated world: from receding sea ice and thawing permafrost to rising temperatures and changes in rain and snowfall.
"I view the decline of the September sea ice in the Arctic as the most conspicuous example of climate change, at least in the ocean," says Thomas Haine, a professor in the Department of Earth and Planetary Sciences whose work has focused largely on changes in the North Atlantic and Arctic. Over the past few decades, summer ice in the Arctic has been in steep decline, falling from about 7 million square kilometers in September 1979 to less than 4 million in September 2020. Winter sea ice continues contracting as well, with the Arctic Ocean now missing a chunk of winter ice coverage the size of Texas and Florida combined, according to NASA. The once-white Arctic Ocean melts to reveal darker water beneath—which in turn absorbs more heat and melts more ice.
Vanishing sea ice because of climate change in the Arctic has been well-documented for at least a decade. But a recent study in the journal Geophysical Research Letters found that increased precipitation—rain and snow—contributes even more to changes in the Arctic Ocean than previously thought. In lower latitudes, humidity evaporates off the ocean, and that moisture is swept through the atmosphere up to the north. The cold holds the moisture captive, making it fall as snow or rain in the Arctic rather than moving it elsewhere. As the globe warms, the strength of that cycle is increasing, Haine says. "The atmosphere is warming up; therefore, it can hold more moisture. And then it carries the moisture it holds over the Arctic, it gets cold, condenses, rains out—either directly onto the ocean or flows in through rivers and streams."
But what happens in the Arctic doesn't stay in the Arctic, as northern observers frequently say. All of that fresh water is released into the North Atlantic Ocean below the Arctic, creating expanses of cold water that rest on the ocean's surface and potentially disrupt ocean circulation. Usually, the AMOC brings warmer water up the East Coast so it can cool in the Arctic and drop down the underwater waterfall. But one such cold blob, formed over a decade ago, is blocking that influx of warm water, causing the circulation to stall.
Such enormous changes have significant effects on ecosystems all along the food chain, from phytoplankton and fish to seals and polar bears—and people. A freshwater buildup means that nutrients below the cold blob no longer mix with it. And in warmer, more acidic waters, entire fisheries could move north or south, or disappear entirely, decimating fishing industries and leaving millions of people hungry. Research published in the journal Science found that up to 60% of fish species could be wiped out by climate change. Polar fish such as Arctic grayling and Dolly Varden char, which support animals and people in the north, could go extinct, research published in the journal Freshwater Biology found. "And so the fisheries collapse," says Gnanadesikan. "There has been some argument made that that was responsible for the collapse of the Newfoundland cod fisheries in the 1990s." More recently, in 2015, Maine's commercial cod fishery collapsed, likely owing to slowing circulation, another study in Science found. Warming waters elsewhere are pushing fish and other marine life northward, but that could spark geopolitical conflict and trade wars as countries grapple with changing resources and fishing quotas.
Haine has focused his research in particular on the Denmark Strait cataract, which is about three times taller than the largest waterfall on land. "Off the southeast coast of Greenland, it starts on a journey through the deep and abyssal ocean that will last hundreds or maybe even thousands of years," Haine says.
He and his team want to understand the exact terrain of the cataract, the density of the cool water flowing down it, and how exactly that turbulent flow works—in order to divine what changes to water temperature and density would mean for this crucial but little-understood link in the AMOC.
It's very difficult and expensive to observe the overflow directly, so much of their work takes place in the lab. And it's much easier and more efficient if they know what they are looking for ahead of time—which is where the highly complex and detailed computer models can help focus their attention.
"The computer models suggest what to look for in the real ocean," Haine says, allowing researchers to make the most of their time while making observations in the field.
He has worked on these models for the past 15 years, and in that time his team has begun noticing patterns in ocean currents that they predict are important but haven't yet been observed in real life. For instance, they believe the water shifts and ebbs and flows, like water glugging out of a bottle, and they suspect that this causes the water to mix shallow and deep water much more than previously thought. The researchers want to design an experiment where they release a dye in the water to see whether the mixing really happens in nature as they see it happening in their computer simulations.
"It opens research opportunities that we've longed to understand for decades, but it has just been impossible to directly observe," Haine says.
While it's clear that climate change is already transforming the ocean in ways humankind has never seen before, researchers hope these models will help them understand exactly how, and how much, it's affecting the waterfall—and, in turn, the AMOC. "We are watching it very carefully, and we're trying to figure out why we see the changes that we see, and what we might expect down the line," Haine says.
Changes to AMOC could have tremendous repercussions for the rest of the world. "The wet areas get wetter and the dry areas get drier," Gnanadesikan says. In 2018, for instance, Arctic sea ice loss led to a historic snowfall in Europe, dubbed "the Beast from the East." The Gulf Stream flowing along the east coast of the Americas usually helps keep temperatures higher in northwest Europe, by conveying warmer air from west to east, but a weakened current could mean more plunging temperatures and snowstorms in Scandinavia and the United Kingdom. Farther south, deserts in Africa could rapidly expand as rainfall becomes scarcer and less reliable.
"Changing AMOC has a big impact on Europe and even on the Sahel," Waugh says. "But once you start changing how much heat and how much carbon you take into the ocean, that'll have a global impact as well."
At the other edge of the world, the wind cuts a deep trough into the Southern Ocean, pushing the surface of the water 6 feet lower than it is in the rest of the globe. The Southern Ocean, in addition to being incredibly cold, is unbelievably windy—and, owing to environmental change, it is getting windier. As the wind howls over the icy expanse of Antarctica, with little blocking its way, it builds up so much speed—up to 70 miles an hour—that it pushes the waves away in both directions. It's like a belt tightening around the bottom of the globe, sending ripples upward. This is the beginning of the ocean currents' journey back up to the tropics, along the shorelines of South and North America, and eventually to the Arctic.
The southern winds drive circulation in the North Pacific as well, but "the places that we think really matter are the Southern Ocean and the North Atlantic," Gnanadesikan says. The North Atlantic is a narrower basin because of the way the continents are shaped, and no deep water forms in the North Pacific as it does in the North Atlantic—which determines these strong circulation patterns.
This continuous push northward also pulls ancient water from deep in the ocean, drawing up the water that was deposited by the undersea North Atlantic waterfall hundreds of years earlier. Gnanadesikan's major contribution to ocean circulation theory has been demonstrating the influence of these winds in the Southern Ocean on overturning circulation around the world. "They are much more effective at bringing cold, deep water up to the surface," he says, than mixing within the ocean from currents or tides.
"Wind-driven circulation is actually responsible for most of the ocean circulation, even what we call the great ocean conveyor. Because it is easy to sink cold water into the deep ocean, but then how do you get it to come back up to the surface? That water down there—what's going to lift it back up?" Gnanadesikan asks. The answer, he's found, is wind. "The Southern Ocean is the engine that drives climate," he says.
But as those winds shift—for instance, by pushing more water south toward Antarctica instead of north to the tropics—the AMOC could also become disconnected at the bottom of the world. The more powerful winds could also push more water to be warmed up north, which Gnanadesikan compares to "keep[ing] the oven door open, pumping more heat into the ocean."
A recent study in the journal Nature Geoscience found, somewhat controversially, that ocean circulation is the weakest it has been in 1,000 years. It was hotly debated, experts say, because historic measurements like these are really hard to make and evaluate, and it's very difficult to account for the ways the ocean changes from season to season and year to year. Because of the immense span of time that water circulates in the ocean, researchers need a similarly long series of measurements in order to make sure a change they're witnessing is actually from climate change, and not just the natural variability of this complex system.
One of the challenges in understanding what's happening in oceans is parsing this natural variability—rises and falls in carbon or heat; temporary changes in circulation—versus changes from anthropogenic climate changes. The AMOC shifts regularly as part of these natural changes; unless researchers actually measure every point, they might think a current has slowed down when it's actually just shifted in a new direction. "We have a really hard time measuring the Atlantic meridional overturning circulation today, with fabulous instruments and fabulous technology," Haine says.
Regardless of its past, the future of AMOC is worrying. The Gulf Stream could weaken by 34% to 45% by 2100, Stefan Rahmstorf, co-author of the Nature Geoscience study and a climate scientist with the Potsdam Institute for Climate Impact Research, told the Pulitzer Prize–winning Inside Climate News. Other studies, such as one published in the journal Nature in 2018, have also found some degree of slowing. The big question is, how much is from climate change and how quickly is the ocean changing?
In order to answer questions like these, scientists need better measurements. Previously, researchers lowered probes into the ocean water, attached to thin copper wires, floating in the ocean's depths until the wires broke. But it's hard to know with certainty exactly which depth they reached—which means the records scientists do have aren't that great. And researchers could only lower a handful of probes on each trip. In 2007, a project named Argo, named for the ship of Greek mythology, was launched with 3,000 robotic "floats" drifting at different depths, measuring water temperature with sensors.
Another way to measure the way currents and circulation work is to examine what's already in the water. Scientists like Gnanadesikan and Waugh measure chemical tracers like radiocarbon, which is produced in the stratosphere by galactic cosmic rays, and chlorofluorocarbons, now-banned compounds once used in refrigerants and aerosols that were pulled into the seawater from the atmosphere. The researchers can then track where the water takes these tracers and compounds, how long the water was at the surface, and what their concentrations are in various parts of the ocean, using those measurements to understand changes in circulation.
Another way to understand what's happening in oceans, and how it is deeply connected to our lives, is examining the geological records of ancient oceans. Gomes is doing exactly that in the Chesapeake Bay. Her research focuses on what the sediment on the ocean floor can tell us about the connections between climate change and decreasing oxygen levels in the ocean. By drilling into the ocean floor with long tubes, called cores, they can examine the sedimentary record—primarily from the Mesozoic, when dinosaurs roamed the earth, flowers evolved, and the poles were ice-free—to understand the series of events that occurred during the past carbon cycle changes.
"We don't really have a good knowledge of what our Earth system is like under rising CO2. So the best way we can really understand what might happen in the future is by looking at the geological record," Gomes says. "What is the threshold over which a CO2 injection is going to have a large effect on the ocean system and then the organisms that live in it?" Gomes asked. The Chesapeake Bay is a case study for the kinds of changes happening in coastal ecosystems around the world—changes that can create more weak links in the ocean's circulation pattern.
When it comes to the ways climate change is affecting ocean ecosystems, Gomes says, "things are already really, really bad."
Studying ancient oceans can help distinguish temporary changes in the ocean from more permanent, human-caused changes from the climate. "We can look back over the last 3 million years and see what the natural variability is in terms of ocean temperature or oxygen availability or other types of proxies," Gomes says. "Just yesterday we hit the CO2 level that is higher than the last 3 million years, so we have to keep on looking back further and further in the geological record to find these time periods."
Many of the major mass extinctions in the rock record are associated with rapid changes in carbon dioxide, Gomes says. "The rate that we are releasing CO2 into the atmosphere is unprecedented, to the best of our ability to measure it. It is a significant problem."
Looking at longtime scales like those measured in the sedimentary record can be reassuring in some ways and alarming in others. Ecosystems find a way to reach a new balance, but that can take thousands of years. "When the environment changes rapidly, things get really dangerous because we can't always adapt on those time scales," Gomes says. And some organisms won't survive. "The planet's going to be fine. It's the organisms on it that are going to have trouble.
"We need to do everything in our power to decrease emissions. But we also have to accept that the climate has already changed, and that we need to figure out how to adapt to it," Gomes says.
Since the beginning of oceanography, the work has relied on physical observations, starting with Captain James Cook using a lead sinker to map ocean depths. Scientists still relied on physical measurements to understand what was happening in the ocean depths right up until the end of the last century. But particularly in the face of complex, interrelated issues like climate change, this work is growing increasingly complicated. Better computer models, driven by the rise in supercomputing, will mean better predictions for what exactly is happening in the ocean, and what it all means moving forward.
Haine first visited the Denmark Strait cataract aboard a Finnish research vessel in 1990 when he was a graduate student studying oceanography. It was fascination at first sight. But soon, he began running up against the limits of direct observation.
Picture the water falling to the deep ocean in the continental divide between Greenland and Iceland. Every day, it's a little different. You can drop an instrument into it, but it won't capture the vast width or depth or volume of this important link in the ocean's circulation.
"The classic way to make measurements on an oceanography ship is you lower an instrument down, you pull it up, you measure temperature, salinity, capture some water," Haine says. "It might take a day, a day and a half, to get maybe 10 or 12 of these profiles. And the entire system would have changed in that time." In a cataract that is 100 kilometers wide and water that is constantly moving and swirling, measurements like these are a drop in the bucket. Computer models, in contrast, offer a three-dimensional view that evolves over time—in the case of these models, usually a year and a half—and cover, in increments of one kilometer at a time, every aspect of the overflow.
Haine's team runs the highest-resolution model of this process out there—but they are always pushing to improve it, to discover more detail, to zoom in as closely as possible. And they want to understand it all now, before these changes start happening, so we have time to understand what it means for the future of the ocean and life on the planet.
Human life is intricately and intimately connected to the ocean in ways we still don't fully understand. But the work of researchers to connect links in these incredibly complex systems could offer a glimpse of the future. It will be up to us to act.
Posted in Science+Technology
Tagged climate change, earth and planetary sciences, oceanography, the ocean issue