Just practicing with the microscope, Audrey Branch knew it was a game-changer. She cried when she saw the first pictures of a mouse brain, its individual neurons glowing red, its spindly dendrites obvious—showing clearly the connections between the cells. Branch, a Kavli Neuroscience Discovery Institute fellow, studies aging by comparing how old and young brain cells connect to form memories—and what might be going wrong with those connections when older people start to forget things. Until recently, getting at that question meant months of tedious preparation of specimens, including slicing each brain small and thin enough to fit under a traditional microscope. And that prep very likely destroyed the delicate connections the Johns Hopkins neuroscientist needed to see.
That changed in April when a new three-dimensional microscope arrived at Johns Hopkins' Homewood campus, a tool that not only condenses what had been months of work into just hours but allows researchers unprecedented views of organs, tissue, and even live specimens. "It's pretty much the greatest thing I've ever experienced in science," Branch says.
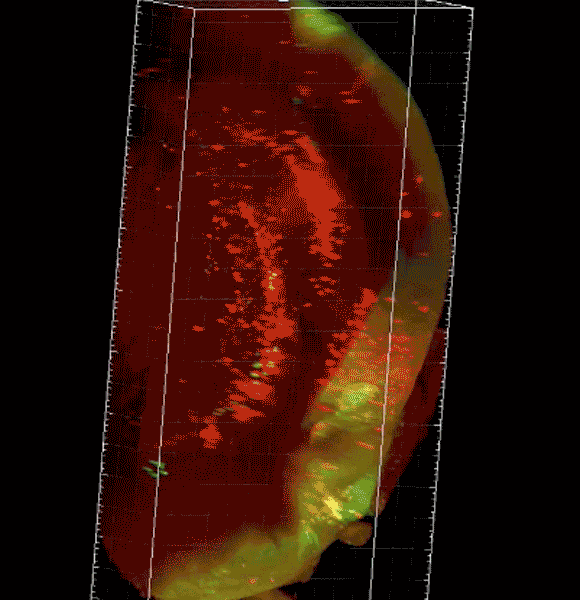
Image caption: The three-dimensional microscope not only condenses what had been months of work into just hours but allows researchers unprecedented views of organs, tissue, and even live specimens.
Purchased with a grant from the National Institutes of Health, the $360,000 instrument—a selective plane fluorescence light sheet microscope—is one of the first in operation on the East Coast and the only one in Maryland. Before researchers can view tissue of any sort with the instrument, their samples must be "cleared," or chemically treated to make them translucent. The microscope then illuminates specimens from the side, shooting two perfectly aligned planes of light across an object, illuminating a wafer-thin slice of the whole while the camera captures the image—thousands of times over as the specimen moves through the light. When the images are displayed together, the result is a three-dimensional image or video clip of the full object, sort of like the more familiar CT scan.
Michael McCaffery, director of the university's Integrated Imaging Center, expects that researchers everywhere will be using this new technology within a few years. In the Johns Hopkins community, word of the light sheet is out and scientists have been lining up to use it, in some cases bringing specimens over from the medical campus. The microscope fills a void, he says. "There was no instrument that allowed a researcher to take a whole organ, brain, or cardiac muscle and image it in three dimensions, in its entirety," McCaffery says.
Most researchers have confocal microscopes, which use lasers to illuminate a sample, point by point, then create computerized images. Confocals produce vivid, high-resolution images, but the sample can't be thicker than a strand of human hair. The light sheet allows samples up to about half an inch in thickness. And because the samples don't have to be cut up, researchers like Branch who are interested in how cells, ducts, or veins connect have a chance to observe them, unspoiled, and create detailed neural maps. "Mapping the neuronal connections of the brain is the holy grail of neurology," McCaffery says.
It certainly is for Branch. Scientists have known that the number of neurons diminishes with age, a process that likely has something to do with why short-term memory degrades with age. But Branch wants to audit the newborn cells in a young brain, determining how many there are, where they are, and what other cells they communicate with. By comparing these cells with those of an older brain, she hopes to see which connections have broken when memory loss occurs. If she can target broken connections, that could provide a way to treat the area with drugs and stop or slow cognitive decline.
Branch has been practicing on the light sheet with mouse brains, but she plans to investigate her hypothesis with rat brains, which are bigger and more humanlike. If she didn't have the light sheet, she would have to slice the rat brain, which is about the size of an olive pit, into about 250 tissue-thin sections. Each slice would then need to be stained, mounted on a slide, and imaged. Finally, she'd have to manually assemble each of those images into a composite to approximate the whole. All of this would take about a month. Since Branch's experiment involves 30 brains, she would need about two and a half years to get through them all, "if," she says, "that's all I did day in and day out." Worse yet, by having to slice the brain, she would likely lose 45 out of 50 marked newborn neurons and all their connections. "I was just going to have to estimate. I'd have missed a lot of the picture, and that's all anyone's been able to do."
Branch expects to have 3-D images of all 30 of her rat brains in three to six months. She'll see each newborn neuron and every dendrite. And hopefully, she'll find answers. She already knows she'll find questions. "It opens up an opportunity to ask even more questions, things that before, we didn't even know we could ask."
Posted in Science+Technology
Tagged neuroscience, kavli institute